Adiabatic frequency conversion using the optical KERR effect
Research
Adiabatic frequency conversion using the optical KERR effect
Demonstration of a low-loss and controllable frequency conversion method
When you pluck a guitar string with your finger, a note of a certain pitch will sound from the guitar for a while. What happens if you quickly change the tension of the string while the note continues to sound? As you can imagine, the pitch of the note emitted from the guitar will change as the tension changes. Physically, this can be explained by the fact that the natural frequency of the string changes as the tension changes.
In fact, a similar phenomenon can also be observed in a micro optical resonator. When the resonant frequency of a micro optical resonator containing light is quickly shifted, the frequency of the light itself in the resonator also changes along with the resonant frequency shift. This phenomenon is known as adiabatic frequency conversion. Until now, most adiabatic wavelength conversion has been achieved by shifting the resonance frequency of a resonator, such as a photonic crystal resonator, through the effect of a carrier. However, the use of carriers has the problems that temporal control is difficult due to the finite diffusion time of the carriers and that the loss of the resonator increases as the carrier density increases. In this study, we realized a low-loss and highly controllable adiabatic frequency conversion by controlling the resonance frequency of a glass resonator called a silica micro optical resonator using the optical Kerr effect, a lossless and instantaneous response technique.
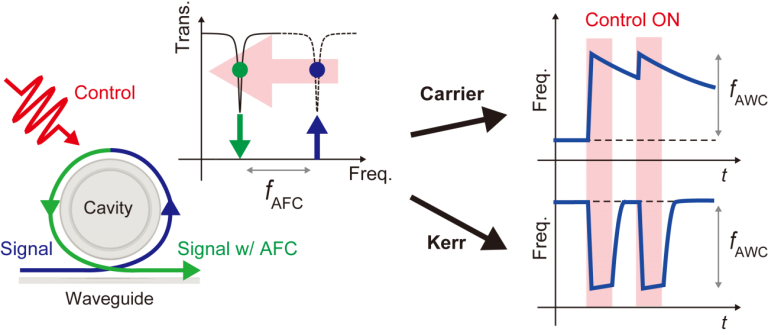
Fig. 1 Schematic of adiabatic frequency conversion. With a carrier, the optical frequency remains converted after the control operation is completed, but with the optical Kerr effect, the frequency can be restored.
Fig. 2 shows the experimental results. If the resonant frequency is not controlled, the optical output should decay smoothly (gray solid line). However, when the resonance frequency is controlled (red region in the figure), oscillations appear in the output (solid blue line). This is a beat caused by the interference effect between the original light and the light in the resonator whose frequency has been shifted, and the beat frequency corresponds to the amount of frequency conversion. In (a), the higher the optical power used to control the resonance frequency, the larger the frequency conversion becomes, reaching a maximum of 140 MHz. In (b), the fast response of the optical Kerr effect is exploited to achieve two conversions. Observation of multiple adiabatic frequency conversions is unprecedented.
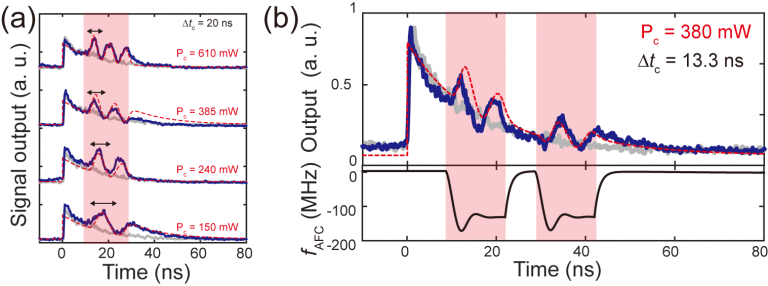
Fig. 2 Experimental results of adiabatic frequency conversion. (a) Power dependence. (b) Multiple operations. The lower panel shows the time variation of the frequency conversion estimated from theory.
The fast response of the optical Kerr effect allows us to investigate the effect of the phase difference between the original and converted light on the output waveform, as shown in Fig. 3(b). This result is in good agreement with the theory. This result is in good agreement with theory.
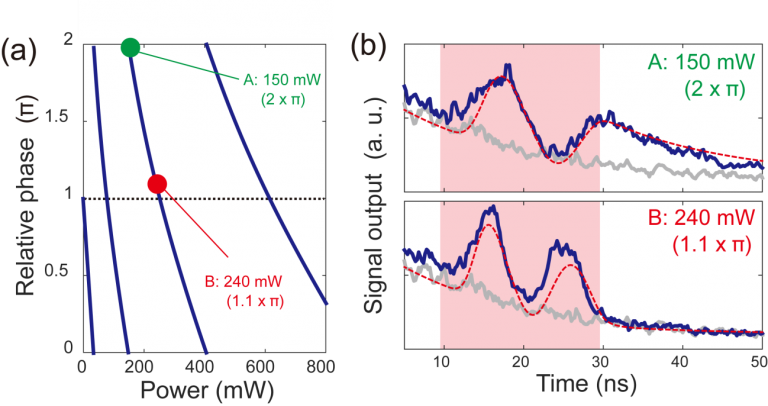
Fig. 3 Analysis of the influence of phase difference. (a) Power dependence of phase difference. (b) Comparison with experimental results. When the phase difference is close to the original value (π) (bottom), the waveform after the conversion operation matches the original waveform. On the other hand, when the phase difference is far from the original value (top), the waveform does not return to the original waveform.
The strength of adiabatic frequency conversion is its ability to smoothly convert frequencies with an efficiency of 100% in principle. Combined with the low-loss property of this research, it is expected to be applied to fields such as quantum information processing. This research has also deepened our understanding of adiabatic frequency conversion.
- Categories
- 研究トピックス